CE Expiration Date: April 20, 2026
CEU (Continuing Education Unit):2 Credit(s)
AGD Code: 010
Educational Aims
This self-instructional course for dentists aims to show that mitochondria are more than just ATP factories. They are now considered central hubs in regulating calcium homeostasis, orchestrating apoptosis, creating chronic inflammation, and regulating innate immunity. Hypoxia and fragmented sleep associated with sleep related breathing disorders promote dysfunctional mitochondria alterations that increase reactive oxygen species which lead to inflammatory processes which contribute to aging and cellular health. This article connects the dots between SRBD and non-communicable diseases, and mitochondrial dysfunction is exposed as the smoking gun. As mitochondria represent the future of medicine, there will always be a seat at that table for SRBD management.
Expected Outcomes
Dental Sleep Practice subscribers can answer the CE questions online to earn 2 hours of CE from reading the article. Correctly answering the questions will demonstrate the reader can:
- Review the components of the eukaryote cell.
- Review the structure and function of mitochondria.
- Learn where and how ROS are formed.
- Understand why triglycerides are increased in SRBD.
- Examine how sleep loss really affects brain function.
- Learn the components of the microbiota-gut-brain-axis.
- Discover how SRBD leads to mitochondrial dysfunction.
- Learn how mitochondrial dysfunction leads to disease.
Dr. Steve Lamberg explains the complicated connection between mitochondria and health. How can this organelle trigger systemic inflammation, accelerated aging, and more? Read about it here.
by Dr. Steve Lamberg
Wouldn’t you love a deeper understanding of the underlying pathophysiology of sleep related breathing disorders “SRBD” and how they cause so many medical conditions? I certainly would. After “peeling this onion” to connect the dots, it’s clear that one’s level of health and wellness is a result of mitochondrial function. A growing body of research reveals that mitochondrial dysfunction is very common and is associated with most chronic disease and early aging. Several recent studies show the intermittent breathing disruptions that characterize obstructive sleep apnea stress mitochondria. The research supports that the free radical production from hypoxic events along with sleep fragmentation are both underlying contributors to mitochondrial dysfunction. The significance of this mitochondrial-SRBD relationship cannot be overstated and should earn it a place in any sleep and airway educational curriculum.
Despite advances in health care, the CDC reports over 60% of US population has at least one chronic disease, and this statistic is trending upward. While scientific literature supports the role of poor breathing and sleeping habits as a causal factor in almost all chronic illnesses, we are just beginning to appreciate that maintaining healthy cellular function is at the core of healthy tissues and organ systems. So how does one go about attaining good cellular function? Current research shows the underlying mechanism by which SRBD cause problems with every one of our body’s systems is by disrupting the normal function of our mitochondria. The necessary balancing act between pro-oxidative productions and a concomitant antioxidant defense systems is referred to as redox homeostasis and is a crucial determinant of physiologic processes to maintain optimal health.1 Although mitochondrial dysfunction can be acquired from aging, trauma, poor diet, and drugs, hypoxia and sleep fragmentation associated with SRBD has recently stolen the limelight. As SRBD is associated with cyclic alterations of arterial oxygen saturation and desaturation as well as sleep fragmentation, these hypoxic and fragmenting events trigger pro-inflammatory processes and interfere with healthy mitochondrial function2 which interferes with healthy cellular function.
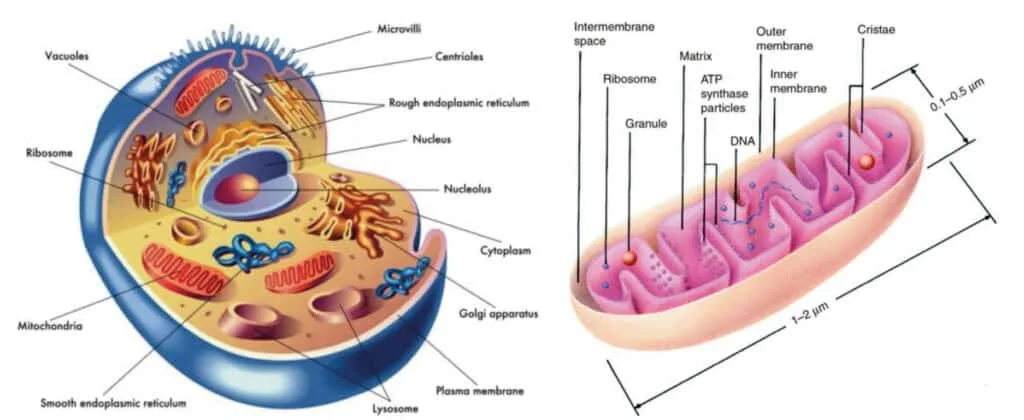
A quick review of the composition of a cell (Figure 1), the structure of the mitochondria (Figure 2), the general energy producing operations (Figure 3), and a more detailed view of the metabolic pathways of energy production (Figure 4) is necessary to appreciate the mitochondrial-SRBD connection.
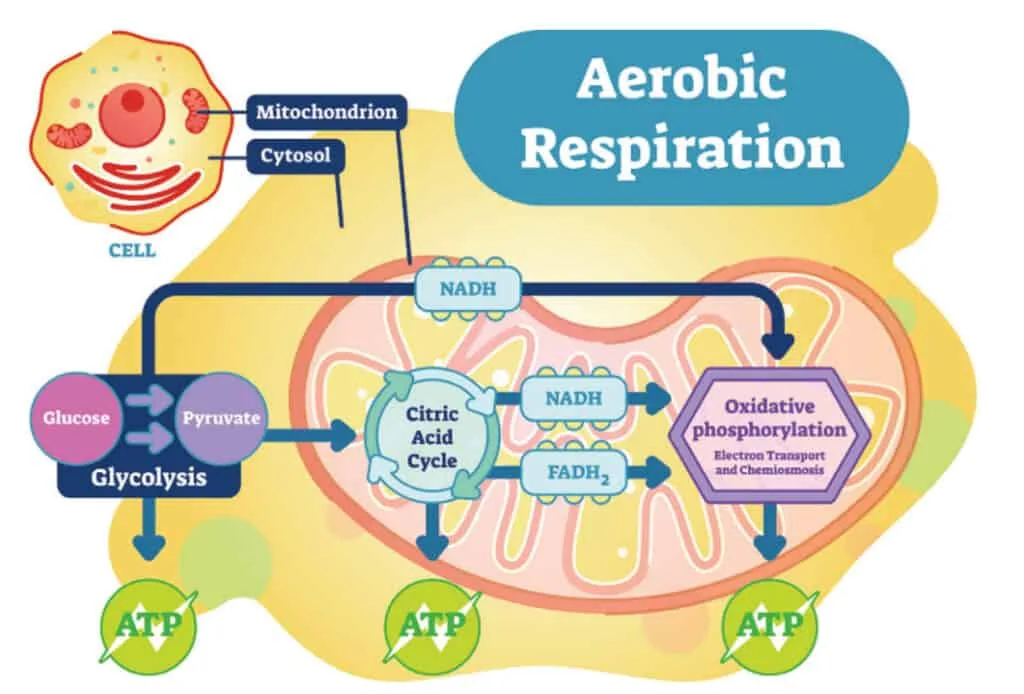
Mitochondria are double membrane cell organelles (Figure 2) which have their own DNA “mDNA” and are primarily tasked with cellular respiration and regulating the metabolic activity of the cell. Evidence shows they evolved from primitive bacteria and each cell has thousands of them. They produce the majority of ATP through the process of oxidative phosphorylation (2 ATP from the Krebs Cycle (also known as the Citric Acid Cycle or the TCA cycle) and 34 ATP from the electron transport chain “ETC”). They can promote cell growth as well as programmed cell death (apoptosis). Additionally mitochondria regulate calcium homeostasis and detoxify ammonia in the liver cells.
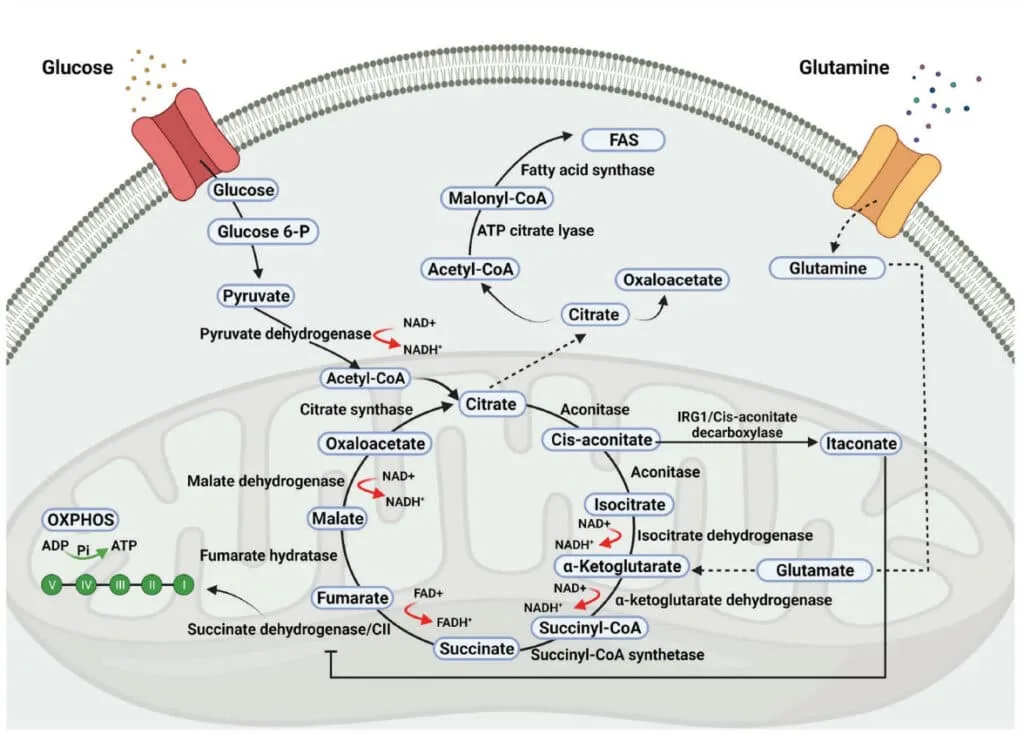
Reactive oxygen species “ROS” are produced in all cell types as byproducts of the mitochondrial election transport chain (ETC) during ATP synthesis (the sequential passage of electrons from high (NADH or FADH2) to low (molecular oxygen), or formed by NADPH oxidase (NOS) in a controlled manner for targeted purposes such as the respiratory burst in macrophages and neutrophils to defend against microbial invasion.4 Sleep fragmentation is inflammatory as well and increases ROS (oxidative stress).
The CDC reports over 60% of the US population has at least one chronic disease, and this statistic is trending upward.
As oxidative stress from the ROS injures the mitochondria, it affects changes in mitochondrial metabolism which trigger systemic inflammation, accelerated aging and apoptosis. Blocking the electron transport chain and fumbling of electrons in the mitochondria result in more oxidation which leads to: accumulation of free radicals, DNA damage accumulation (deletions and mutations) leading to chronic inflammation, damage to lipids, damage to proteins, and increase in mitochondrial membrane permeability. Mitochondria DNA “mDNA” deletions cause major disruption of metabolism and can result in severe cellular impairment or death.5
ROS has another important job as it is linked to the hypoxia-inducible factor 1 “HIF-1” system and it allows anticipating multiple layers of reciprocal interaction to help the mitochondria adapt to hypoxia. The HIF-1 system regulates mitophagy – the reduction of mitochondrial mass by removal of damaged mitochondria.6
In SRBD, mitochondrial dysfunction as a result of redox imbalance can prevent pyruvate, a byproduct of glycolysis, from even entering the mitochondria and leads instead to the pyruvate being converted into the fatty acid palmitic acid (“de novo lipogenesis”), which in turn is exported from the liver and increases triglyceride levels.7 This has a tendency to hinder glucose uptake into the cells which can lead to insulin resistance and T2DM.
Sleep and wakefulness can affect changes in mitochondrial gene expression, oxidative phosphorylation activity, and morphology in the brain.8 Sleep loss affects brain function in numerous ways including disrupting both short and long-term memory, attention, decision making, and has even been proposed as a trigger for manic episodes in bipolar disorder in humans. Morphological changes to neuronal mitochondria have been reported after prolonged sleep deprivation “SD”. Morphological changes relate to SD-driven changes in mitochondrial cristae functions such as cytochrome c storage or ETC activity. Taken together the reported effects of SD on mitochondrial morphology suggest a major impact on neuronal energy production, and potentially also on neuronal viability. In fact, available data suggest that sleep loss disrupts such fundamental cellular processes as transcription, translation, intracellular transport, and metabolism.9
The regulation of hypoxia has recently emerged as having a central role in mitochondrial function and dysfunction in various diseases, including the major disorders worldwide: CVD and cancer. Accumulating evidence demonstrates that mitochondria are the major target of hypoxic injury, the most common source of ROS during hypoxia, and are the key elements for inflammation regulation during the development of both CVDs and cancer. Taken together, observations propose that hypoxia, mitochondrial abnormality, oxidative stress, inflammation in CVDs, and cancer are closely linked.10
Oxidative stress and mitochondrial damage have also been implicated in pathogenesis of several neurodegenerative diseases, including Alzheimer’s disease, Parkinson’s disease, and amyotrophic lateral sclerosis.11
Abnormalities in mitochondrial metabolism have also been shown to contribute to hypoxia-induced placental oxidative stress. Intermittent hypoxia resulted in reductions in mitochondrial content, decreased abundance of key molecules involved in the electron transport chain and increased expression and activity of glycolytic enzymes. Expression levels of key regulators of mitochondrial biogenesis were decreased while the abundance of constituents of mitophagy, autophagy and mitochondrial fission machinery was increased in response to hypoxia. Placental hypoxia was associated with mitochondrial-generated ROS, increased oxidative stress, alterations in molecular pathways controlling mitochondrial content and function, inflammation, and apoptosis.12
The microbiota-gut-brain axis is a complex multidirectional cross-talk system between the gut microbiota, the enteric nervous system, and the brain. It acts as an adaptive interface with the environment. A strong interplay also exists with the neuroendocrine-immune network and therefore, the functional integrity of this axis is required for the homeostasis of several systems.13 Mitochondria emerged from bacterial ancestors during endosymbiosis. Mitochondria and bacteria had analogous genomic characteristics, similar bioactive compounds, and comparable energy metabolism pathways. The interactions of intestinal microbiota with neural mitochondria have become an area of investigation. Recent studies have identified neural mitochondrial dysfunction as a critical pathogenic factor for the onset and progress of multiple neurological disorders, in which the role of altered gut flora composition was increasingly noticed. It has been proposed that the communicating channel from gut to brain involves the interaction between intestinal microbiota and neural mitochondria.14 As mitochondria supply the necessary energy and messaging capacity to maintain this complex neuronal network, any mitochondrial dysfunction that results from oxidative stress in SRBD will have a negative impact on the overall health of this system. Emerging evidence suggests that gut microbiota also communicate with the mitochondria of mucosal cells, including epithelial cells and immune cells. Gut microbiota signaling to mitochondria has been shown to alter mitochondrial metabolism, activate immune cells, induce inflammasome signaling, and even alter epithelial barrier function. Both dysbiosis of the gut microbiota and mitochondrial dysfunction are associated with inflammatory bowel disease “IBD” and colorectal cancer.15
Another area of interest is the role of mitochondria in skeletal muscle tissue in obesity. Recent studies reveal that disorder in the balance between mitochondrial fission and fusion is implicated in mitochondrial damage and dysfunction in skeletal muscle in obesity. Genioglossus injury in obese mice that were fed a high fat diet was mediated by mitochondrial disturbance.16
A short list of pro-mitochondrial health suggestions include:
- Eat a balanced diet which includes healthy fats such as omega-3s and avoid processed foods and refined carbohydrates which interfere with mitochondrial molecular pathways. Restrict calories and try to eat within a 10-hour window.
- Maintain a daily exercise routine that includes high intensity interval training that includes aerobic activities like swimming biking and hiking.
- Prioritize getting 7 hours of quality sleep. Sleep related breathing disorders cause hypoxia and sleep fragmentation, both of which compromise mitochondrial health by increasing ROS which leads to chronic inflammation.
- Supplementation can reduce oxidative stress and stimulate mitochondrial health. Melatonin and αlpha-lipoic acid are strong mitochondrial antioxidants. Resveratrol is another antioxidant which is found in red wine and dark chocolate, however you would get rather drunk and fat getting your therapeutic dosing this way. Stick to the inexpensive pill. Caffeine increases mitochondrial function.
- Heat therapy from a sauna or steam bath has been shown to increase the efficiency of mitochondria. Be vigilant about electromagnetic fields “EMFs” which are prevalent in some far infrared saunas. EMFs have been shown to cause mitochondrial stress and dysfunction which will easily outweigh any benefits.
- Practice relaxation techniques like meditation and massage to reduce stress hormones such as cortisol which negatively effects immune, nervous, and endocrine systems. Simply hiking in nature has been shown to decrease amygdala activity.
This “mitochondriac journey” is not just for your patients, it’s for you too! Look at your own concept of wellness and illness. Reboot your own mitochondria to prevent chronic degenerative disease before it becomes irreversible! Where there are healthy mitochondria there is absence of disease.
Understanding the mechanisms by which SRBD cause mitochondrial dysfunction, and eventually disease, will hopefully provide health care providers with therapeutic targets as solutions to optimize mitochondrial health and therefore total body health. “Great things are done by a series of small things brought together.” – Vincent Van Gogh
References
- Ziqing Li , Dongliang Xu, Xinhua Li, Yilun Deng, and Chaohong Li, Redox Imbalance in Chronic Inflammatory Diseases, Hindawi, BioMed Research International Volume April 2022, Article ID 9813486
- Lacedonia et al. Mitochondrial DNA alteration in obstructive sleep apnea. Respiratory Research (2015) 16:47
- Schroder, “NADPH oxidases in bone homeostasis and osteoporosis,” Free Radical Biology & Medicine, vol. 132, pp. 67– 72, 2019.
- Levy P, Tamisier R, Arnaud C, Monneret D, Baguet JP, Stanke-Labesque F, et al. Sleep deprivation, sleep apnea and cardiovascular diseases. Front Biosci. 2012;1(4):2007–21.
- Elizabeth Murphy, Hossein Ardehali, Robert S. Balaban, Fabio DiLisa, Gerald W. DornII, Richard N. Kitsis, Kinya Otsu, Peipei Ping, Rosario Rizzuto, Michael N. Sack, Douglas Wallace and Richard J. Youle, Mitochondrial Function, Biology, and Role in Disease – A Scientific Statement From the American Heart Association, Circulation Research. 2016;118:1960–1991
- Fuhrmann, Brune, Mitochondrial composition and function under the control of hypoxia, Redox Biology, 12 (2017) 208-215
- Kollar et al. The impact of sleep apnea syndrome on the altered lipid metabolism and the redox balance, Lipids in Health and Disease (2021) 20:175
- Kim et al: Increased mtDNA Copy Number in the Rat Hippocampus After REM-Sleep Deprivation in vivo 36: 1726-1733 (2022)
- Lijing Wang and Sara J. Aton, Perspective – ultrastructural analyses reflect the effects of sleep and sleep loss on neuronal cell biology, SLEEPJ, 2022, Vol. 45, No. 5
- Bouhamida E, Morciano G, Perrone M, Kahsay AE, Della Sala M, Wieckowski MR, Fiorica F, Pinton P, Giorgi C, Patergnani S. The Interplay of Hypoxia Signaling on Mitochondrial Dysfunction and Inflammation in Cardiovascular Diseases and Cancer: From Molecular Mechanisms to Therapeutic Approaches. Biology (Basel). 2022 Feb 12;11(2):300.
- Giovanna Cenini, Ana Lloret , and Roberta Cascella, Review Article: Oxidative Stress in Neurodegenerative Diseases: From a Mitochondrial Point of View, Hindawi, Oxidative Medicine and Cellular Longevity Volume 2019, Article ID 2105607
- Vangrieken P, Al-Nasiry S, Bast A, Leermakers PA, Tulen CBM, Janssen GMJ, Kaminski I, Geomini I, Lemmens T, Schiffers PMH, van Schooten FJ, Remels AHV. Hypoxia-induced mitochondrial abnormalities in cells of the placenta. PLoS One. 2021 Jan 12;16(1)
- Laura Dumitrescu, Iulia Popescu-Olaru, Liviu Cozma, Delia Tulbă, Mihail Eugen Hinescu , Laura Cristina Ceafalan ,Mihaela Gherghiceanu and Bogdan Ovidiu Popescu, Oxidative Medicine and Cellular Longevity, Hindawi, Volume 2018, Article ID 2406594
- Zhu Y, Li Y, Zhang Q, Song Y, Wang L and Zhu Z, Interactions Between Intestinal Microbiota and Neural Mitochondria: A New Perspective on Communicating Pathway From Gut to Brain. Front. Microbiol. (2022) 13:798917
- Dakota N Jackson , Arianne L, Theiss Gut bacteria signaling to mitochondria in intestinal inflammation and cancer, 2020 May 3;11(3):285-304.
- Chen, Han, Chen, Zhao, Sun, Sun ,Zhang, Yu, Lui, High-Fat Diet-Induced Mitochondrial Dysfunction Promotes Genioglossus Injury – A Potential Mechanism for Obstructive Sleep Apnea With Obesity, Nature and Science of Sleep 2021:13 2203-2219